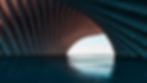
The ages of civilisation have been determined by the mastery of new materials.
We are now entering an era where it is possible to design materials from the molecular level upwards.
This gives the potential for staggering improvements in performance.
Our mission is to conduct new molecular-level design and development of materials
We are able to achieve materials with ultra high toughness, fire resistance, thermal conductivity, as well as materials derived from sustainable ingredients
We use an agile approach to materials science
Undertaking a broad range of R&D activities
Cutting Edge Research
World leading research into new materials
Rapid Prototyping of Materials and Designs
One week material development cycles
Extensive Testing Capabilities
From battery cycling to full aerospace regulation tests
Novel Materials Database
We have characterised thousands of novel materials
Advanced Modelling
From molecular modelling to finite element methods